Exploring the drivers of modern global warming
Posted on 8 January 2025 by Zeke Hausfather
This is a re-post from the Climate Brink
Global surface temperatures have risen around 1.3C since the preindustrial (1850-1900) period as a result of human activity. However, this aggregate number masks a lot of underlying factors that contribute to global surface temperature changes over time.
These include CO2, which is the primary driver of long-term warming, as well as non-CO2 greenhouse gases like CH4, N2O, and halocarbons. But it also includes planet-cooling aerosols that have masked a sizable portion of the warming of our greenhouse gas emissions to-date. Rounding out the list are other anthropogenic factors (tropospheric ozone, albedo changes due to land use change), and natural forcings (primarily volcanic eruptions and variations in solar output).
To disentangle the respective contributions of each of these requires a climate model. Here I will be using the latest version of FaIR, a reduced complexity climate model that has been used extensively by the community for assessing global-level changes. The implementation of FaIR used here is specifically designed to reproduce both observed climate change since pre-industrial and assessed climate metrics from the IPCC Sixth Assessment Report (AR6). This approach has the advantage of providing robust uncertainties that reflect the range of relevant parameters (e.g. climate sensitivity, carbon cycle feedback strength, ocean heat uptake rates, etc.) in-line with the ranges in the AR6.
However, unlike the climate simulations featured in the AR6, which only use climate forcings based on real-world observations through 2014 and explore different scenarios (SSPs) thereafter, these simulations use observationally-informed forcing estimate through the end of 2023 from Forster et al 2024. This has the advantage of allowing us to explore how actual changes in real-world emissions (e.g. including factors like rapid Chinese aerosol declines and low-sulfur shipping rules) have impacted global temperatures.
The figure below shows the output of 841 different emission-driven FaIR model runs across the range of constrained parameter values. It simulates both the global surface temperature response to all forcings (in yellow), as well as estimates of each forcing in isolation (calculated by comparing the difference between runs containing all forcings and those excluding one type of forcing).
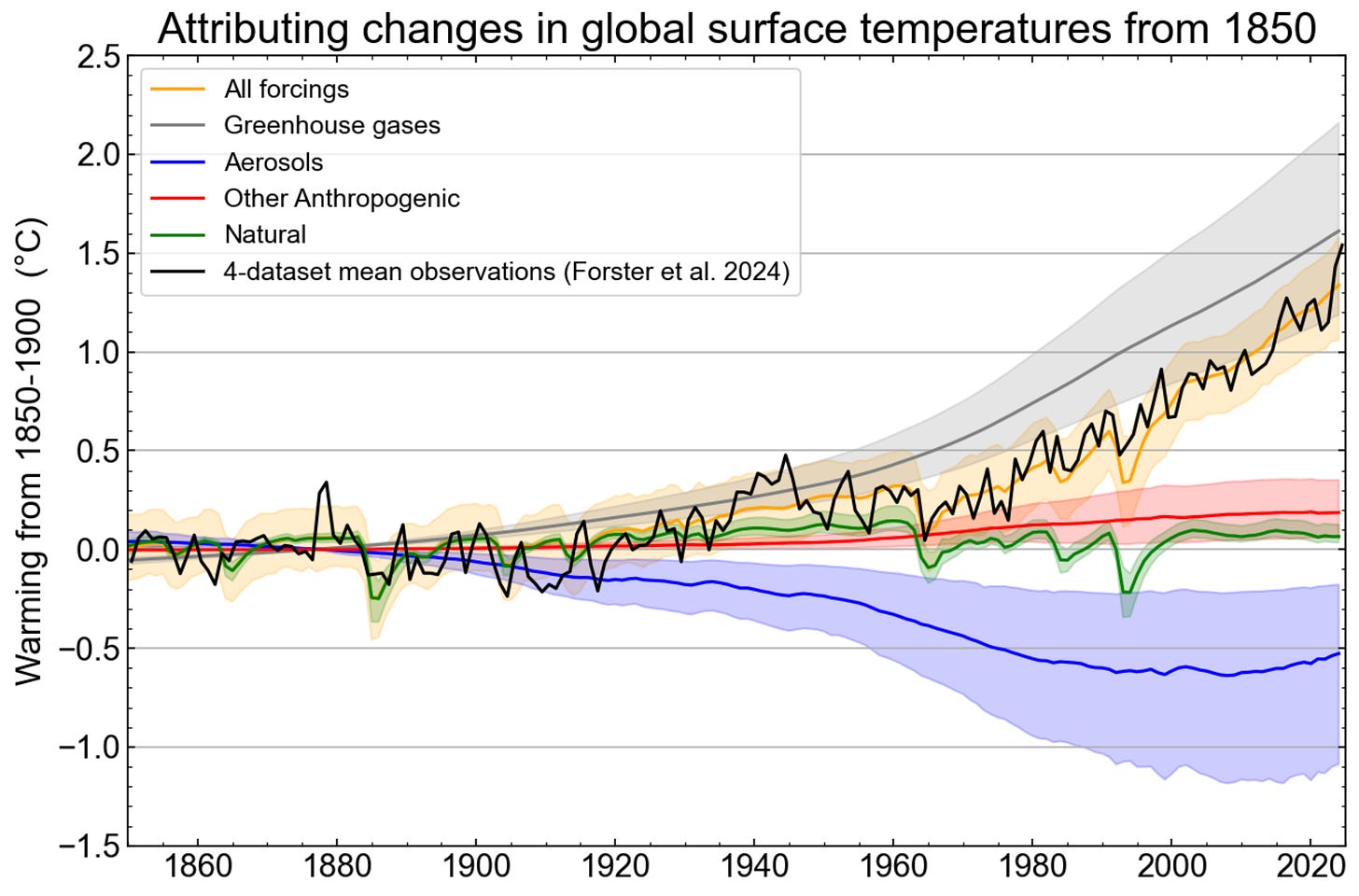
Observed global surface temperatures since 1850 (black), along with modeled temperatures (yellow) and separate temperature components (other colors).
Note that while the uncertainties of some individual elements (e.g. greenhouse gases and aerosols) are larger than those of all forcings, some of these cancel out in the aggregate estimate due to correlations between the two. For example, scenarios with higher warming from greenhouse gas emissions also tend to have higher cooling from aerosols.
The black line in the figure shows observed global surface temperatures. While it generally remains within the range of uncertainty in the model estimate, there is significant year-to-year natural variability driven primarily by El Nino and La Nina events that is not present in the average of all the FaIR runs.
Having simulated the drivers of warming over time, we can use this data to explore in more details the drivers of changes in global temperatures over different time periods. For simplicity I will be showing the median (50th percentile) estimate of the contribution of each factor, but this approach could easily be used to show uncertainties as well.
First, lets look at what is driving changes between preindustrial (~0C) and 2024 (~1.3C). This is more or less whats being shown between the beginning and end of the prior graph, but broken down into a bit more detail. Specifically, I’m separating out methane (CH4) from other greenhouse gases, and ordering the bars based on the size of their (positive) impact on global temperatures.
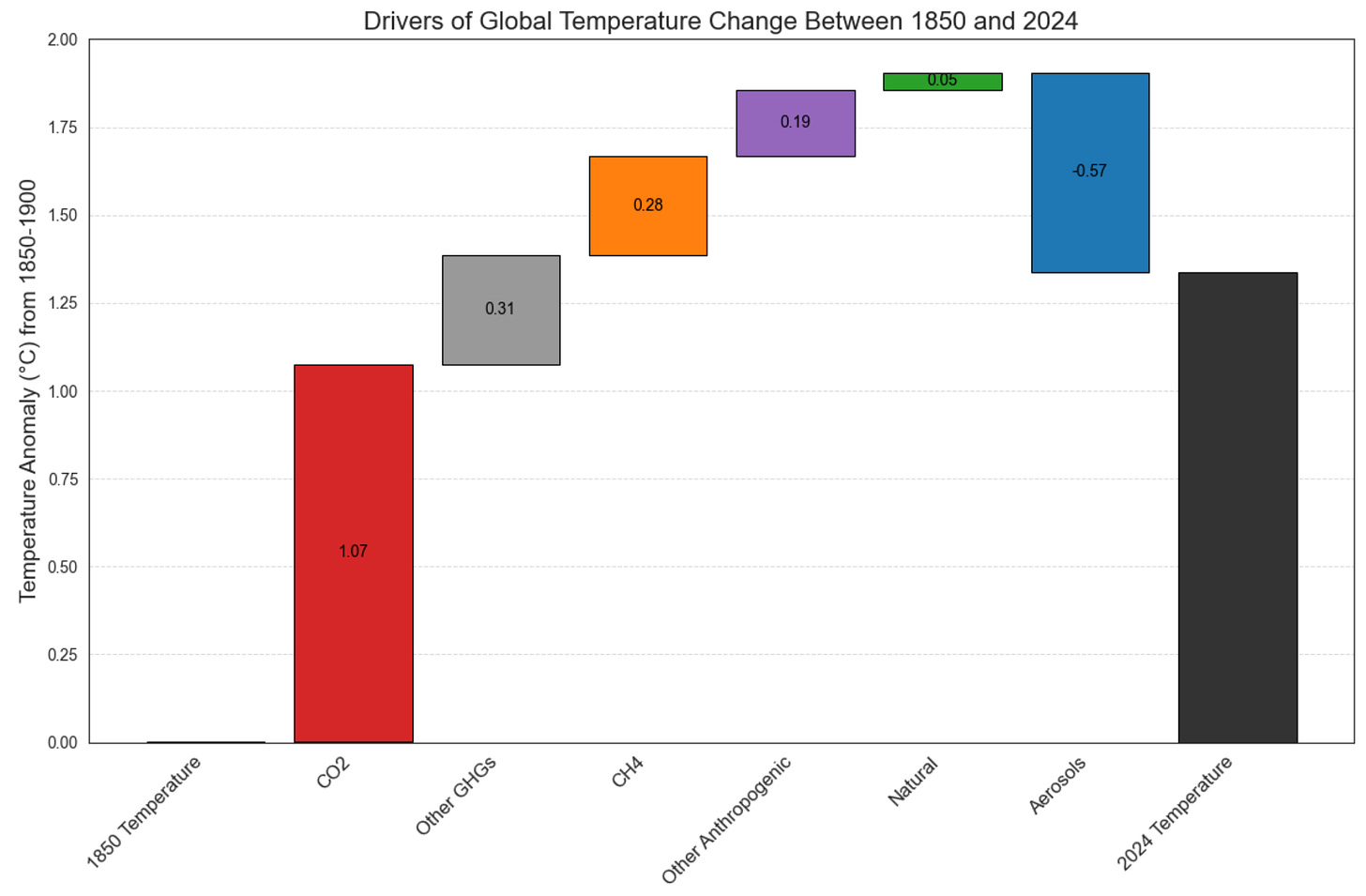
Waterfall diagram showing components of global temperature change between 1850 and 2024.
Here we see that since 1850, CO2 has contributed around 1.1C warming, CH4 contributed ~0.3C warming, other greenhouse gases ~0.3C warming, and other anthropogenic factors ~0.2C. Aerosols have cooled the climate by around 0.6C, while natural variability has only warmed the planet by around 0.05C.
If we look at the “modern warming period” after 1970, the role of methane warming and aerosol cooling are relatively smaller, as both atmospheric methane concentrations and aerosol emissions increased more rapidly in the earlier parts of the century than in the period since 1970.
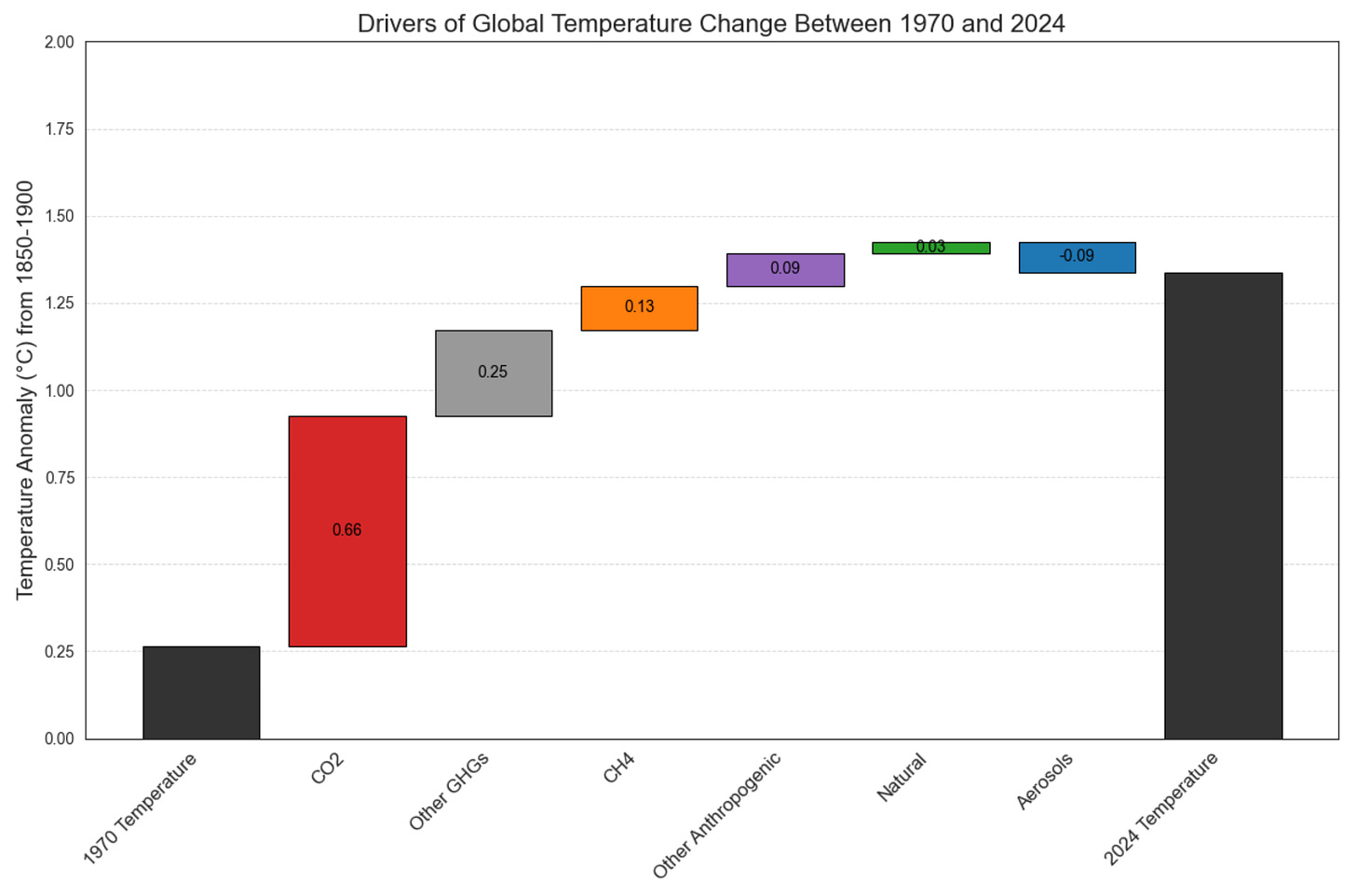
Components of global temperature change between 1970 and 2024.
Since 1970, CO2 contributed ~0.7C warming, other greenhouse gases ~0.3C, CH4 ~0.1C, and other anthropogenic forcings ~0.1C. Aerosols cooled the climate by around 0.1C, while and natural variability had effectively no impact on the difference between 1970 and 2024 temperatures.
Finally, we can look at the drivers of warming the world has experienced over the past decade (2015-2024). Note that the y-axis in the figure below is narrower than in the prior two graphs to zoom in on changes over the past decade, and the axis does not begin at zero.
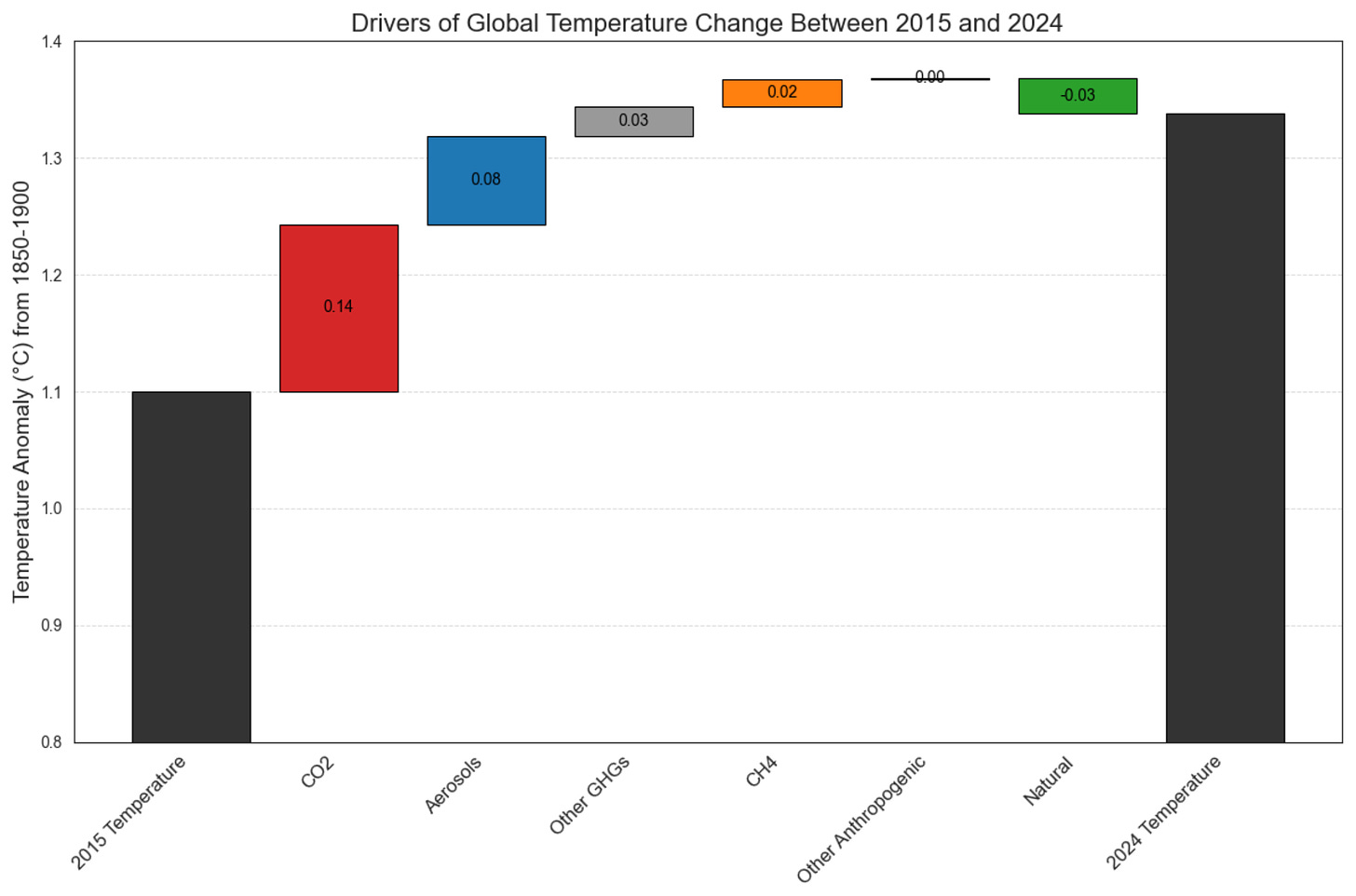
Components of global temperature change between 2015 and 2024.
Over the past decade, CO2 contributed the bulk of greenhouse gas warming at 0.14C, with other greenhouse gases, CH4, and other anthropogenic collectively only warming the planet 0.05C. Due to rapid declines in emissions of planet cooling aerosols (due in large part to declining sulfur emissions from China and international shipping), the planet warmed by around 0.06C over the past decade from greenhouse gas warming previously masked by aerosols. Finally, natural variability had a small (0.03C) cooling effect on the past decade due to the timing of volcanic eruptions and the solar cycle.
While the climate forcings modeled here affect long-term temperature changes, the temperatures we observe in the real world are also effected by El Nino, La Nina, and other modes of short-term natural variability. This does not add any net energy to the Earth system – and thus cannot drive long-term climate changes – but does have a large impact on individual years or even decadal-scale trends (e.g. in cases where a decade starts or ends with a strong El Nino or La Nina event).
In addition to looking at changes between any two years, we can also use these climate model runs to asses the rate of warming over time (excluding unforced short-term variability).
The figure below shows the rate of warming (in degrees C per decade) for each 10-year period between 1905-1914 and 2015-2024. I’ve initially excluded natural forcings from the figure as they result in large changes in decadal trends around major volcanic eruptions.
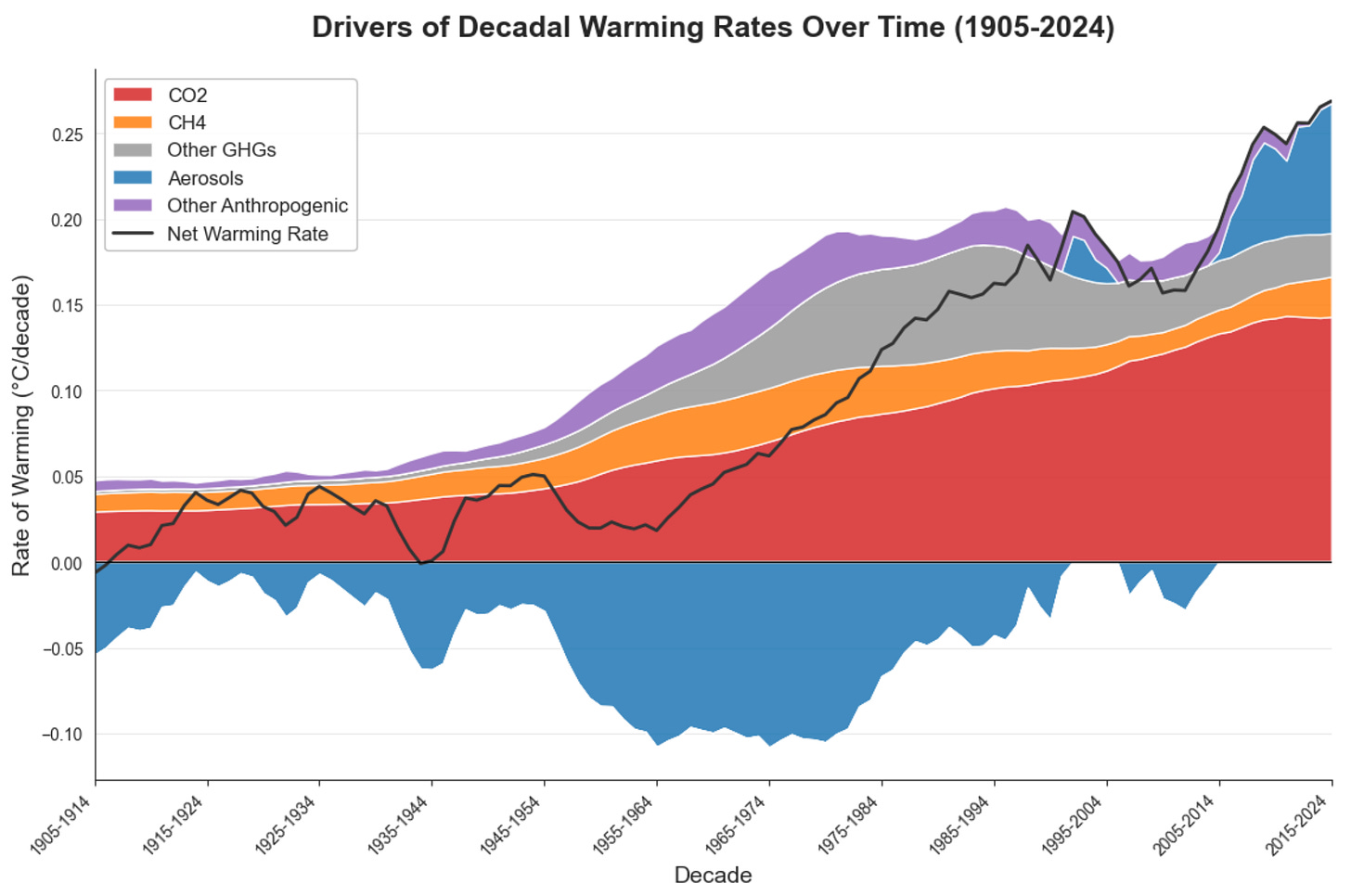
Analysis of decadal warming rates (black line) as the sum of different contributing factors (colored areas) between 1905 and 2024.
We can draw a few interesting conclusions from this figure:
-
The rate of warming increased between the start of the century and 1970, remained relatively flat at just below 0.2C per decade from 1970 to 2005, and has accelerated to ~0.25C per decade over the past decade.
-
The primary driver of this recent acceleration in warming has been declining aerosol emissions. Aerosols have flipped from reducing the rate of decadal warming (as emissions increased) to increasing the rate of warming (as emissions decreased) after 2005.
-
The rate of warming from CO2 has increased over time as emissions have increased, though it has plateaued over the past decade as CO2 emissions have plateaued. However, warming from all greenhouse gas emissions (CO2, CH4, and others) has been relatively flat since 1970. This is primarily due to the declining contribution of other greenhouse gases to additional warming, likely associated with the phaseout of halocarbons after the Montreal protocol.
Its important to note that this figure shows the rate of warming rather than total warming (as the prior figures have shown). A slowdown in the rate of warming from a specific forcing (e.g. halocarbons post-Montreal or CH4 in the 1990s-2010s) still means that the total amount of warming forcing is increasing over time, just more slowly.
Finally, the figure below shows the drivers of decadal warming including natural forcings (e.g. volcanoes and solar output). Volcanoes create a saw-tooth pattern in the resulting decadal warming rates, suppressing the rate of warming in decades that end with the volcanic eruption (and its associated short-term cooling) and enhancing the rate of warming in decades that start out cool and recover from the eruption.
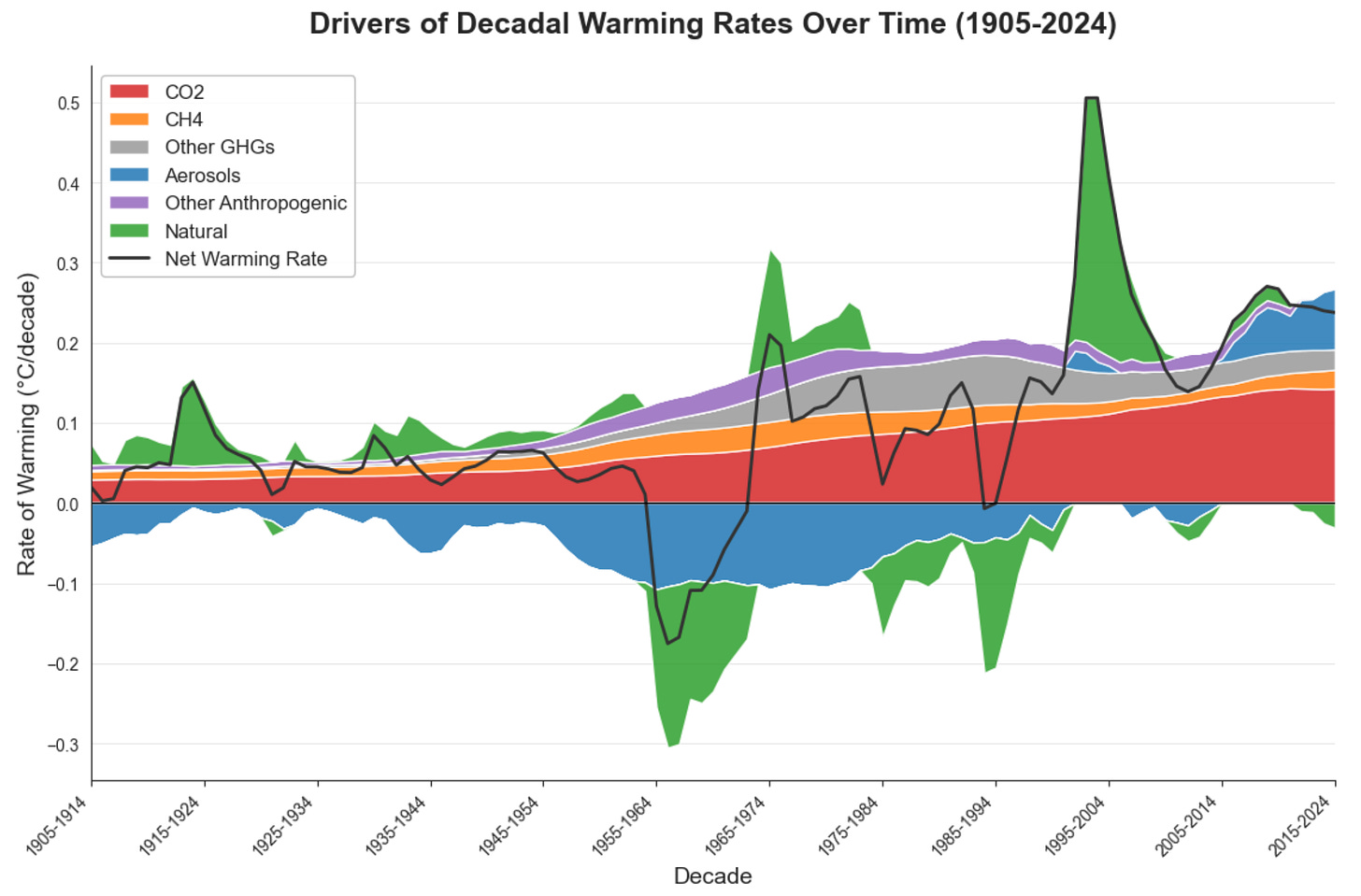
Same as the prior figure, but including natural variability.